Visnyk of the Lviv University. Series Physics
58 (2021) ñ. 98-107
DOI: https://doi.org/10.30970/vph.58.2021.98
Luminescence of CsPbBr3 nanoparticles embedded in KBr
M. P. Dendebera, T. C. Malyi, A. C. Pushak, Ya. M. Chornodolskyy, O. T. Antonyak, V. V. Vistovskyy, A. S. Voloshinovskii
| 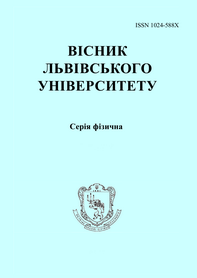 |
CsPbBr3 nanoparticles embedded in KBr single crystal are obtained by adding lead (II) bromide (PbBr2) and cesium bromide (CsBr) salts in the required ratio to KBr. The crystals were grown by the Bridgman method. During the growth of KBr crystals with CsBr and PbBr2 impurities, CsPbBr3 nanocrystals and microcrystals are formed as a result of diffusion of Cs+ and Pb2+ cations. The KBr-CsPbBr3 samples had the impurity content of (CsBr and PbBr2) 0.05, 0.075, 0.2 0.5 1 and 2 mol.{\%} in the KBr matrix. At concentrations less than 1 mol.{\%}, the preferred phase is CsPbBr3 nanoparticles. For higher concentrations, the probability of CsPbBr3 microcrystals formation increases significantly.\\
The ensemble of CsPbBr3 nanoparticles is formed with a certain average size and consequently the spectra shape partially reproduces some size distribution of these nanoparticles. The shift of exciton emission band to the lower wavelength region with decreasing nanoparticle concentration in KBr matrix due to quantum confinement was observed. The halfwidth of exciton luminescence band was used to estimate the average nanoparticle size, which was 7 nm for 0.05 mol.{\%} and 20 nm for 1 mol.{\%} of CsPbBr3.\\
The nature of the exciton emission of nanoparticles can be explained by using the exciton model, which takes into account the exchange interaction and the existence of triplet and singlet excitons. According to this model the observed decrease of the decay time constant with decreasing nanoparticle size is due to the increase of exchange interaction parameter – the potential barrier between singlet and triplet states. Another exciton model takes into account the existence of direct and indirect excitons. In the ensemble of nanoparticles, especially at room temperature, the bands corresponding to direct and indirect exciton emission are overlapping. In addition the size distribution of nanoparticles also effects the half-width of luminescence band.\\
The decrease of luminescence decay time constant with decreasing of nanoparticle size can be explained by the influence of the energy transfer processes between nanoparticles, as well as the increase of the exchange interaction with nanoparticle size decreasing. The difference in the decay kinetics for different emission bands corresponding to nanoparticles with a certain average size is rather due to the increase of exchange interaction with significant decreasing of nanoparticle sizes.
Full text (pdf)
References
- L. Protesescu, S. Yakunin, M.I. Bodnarchuk, F. Krieg, R. Caputo, C.H. Hendon, R.X. Yang, A. Walsh, and M. V. Kovalenko, Nano Lett. 15, 3692 (2015). Doi:0.1021/nl5048779.
- M. V. Kovalenko, L. Protesescu, and M.I. Bodnarchuk, Science. 358, 745 (2017). Doi:10.1126/science.aam7093.
- X. Li, Y. Wu, S. Zhang, B. Cai, Y. Gu, J. Song, and H. Zeng, Adv. Funct. Mater. 26, 2435 (2016). Doi:10.1002/adfm.201600109.
- I. Levchuk, A. Osvet, X. Tang, M. Brandl, J.D. Perea, F. Hoegl, G.J. Matt, R. Hock, M. Batentschuk, and C.J. Brabec, Nano Lett. 17, 2765 (2017). Doi:10.1021/acs.nanolett.6b04781.
- T.M. Demkiv, S. V. Myagkota, T. Malyi, A.S. Pushak, V. V. Vistovskyy, P.M. Yakibchuk, O. V. Shapoval, N.E. Mitina, A.S. Zaichenko, and A.S. Voloshinovskii, J. Lumin. 198, 103 (2018). Doi:10.1016/j.jlumin.2018.02.021.
- P. Li, W. Xie, W. Mao, Y. Tian, F. Huang, S. Xu, and J. Zhang, J. Mater. Chem. C 8, 473 (2020). Doi:10.1039/c9tc06075h.
- M. Nikl, K. Nitsch, K. Polak, G.P. Pazzi, P. Fabeni, D.S. Citrin, and M. Gurioli, Phys. Rev. B 51, 5192 (1995). Doi:10.1103/PhysRevB.51.5192.
- R. Aceves, V. Babin, M.B. Flores, P. Fabeni, A. Maaroos, M. Nikl, K. Nitsch, G.P. Pazzi, R.P. Salas, I. Sildos, N. Zazubovich, and S. Zazubovich, J. Lumin. 93, 27 (2001). Doi:10.1016/S0022-2313(01)00175-2.
- M. Nikl, K. Nitsch, E. Mihokova, K. Polak, P. Fabeni, G.P. Pazzi, M. Gurioli, S. Santucci, R. Phani, A. Scacco, and F. Somma, Phys. E Low-Dimensional Syst. Nanostructures 4, 323 (1999). Doi:10.1016/S1386-9477(99)00016-8.
- S. Myagkota, A. Gloskovskii, R. Gladyshevskii, A. Voloshinovskii, and P. Rodnyi, J. Phys. Condens. Matter 16, 483 (2004). Doi:10.1088/0953-8984/16/3/024.
- M. P. Dendebera, Ya. M. Chornodolskyy, A. V. Zhyshkovych, V. M. Salapak, N. E. Mitina, R. V. Gamernyk, V. V. Vistovskyy, A. C. Voloshinovskii, Visnyk of the Lviv University. Series Physics 56 122 (2019). Doi:10.30970/vph.56.2019.122.
- Q.A. Akkerman, S.G. Motti, A.R. Srimath Kandada, E. Mosconi, V. D’Innocenzo, G. Bertoni, S. Marras, B.A. Kamino, L. Miranda, F. De Angelis, A. Petrozza, M. Prato, and L. Manna, J. Am. Chem. Soc. 138, 1010 (2016). Doi:10.1021/jacs.5b12124.
- X. Yuan, P. Jing, J. Li, M. Wei, J. Hua, J. Zhao, L. Tian, and J. Li, RSC Adv. 6, 78311 (2016). Doi:10.1039/c6ra17008k.
- M.A. Becker, R. Vaxenburg, G. Nedelcu, P.C. Sercel, A. Shabaev, M.J. Mehl, J.G. Michopoulos, S.G. Lambrakos, N. Bernstein, J.L. Lyons, T. Stoferle, R.F. Mahrt, M. V. Kovalenko, D.J. Norris, G. Raino, and A.L. Efros, Nature 553, 189 (2018). Doi:10.1038/nature25147.
- L. Chen, B. Li, C. Zhang, X. Huang, X. Wang, and M. Xiao, Nano Lett. 18, 2074 (2018). Doi:10.1021/acs.nanolett.8b00184
- M. Dendebera, Y. Chornodolskyy, R. Gamernyk, O. Antonyak, I. Pashuk, S. Myagkota, I. Gnilitskyi, V. Pankratov, V. Vistovskyy, V. Mykhaylyk, M. Grinberg, and A. Voloshinovskii, J. Lumin. 225, 117346 (2020). Doi:10.1016/j.jlumin.2020.117346.
- B. Wu, H. Yuan, Q. Xu, J.A. Steele, D. Giovanni, P. Puech, J. Fu, Y.F. Ng, N.F. Jamaludin, A. Solanki, S. Mhaisalkar, N. Mathews, M.B.J. Roeffaers, M. Gratzel, J. Hofkens, and T.C. Sum, Nat. Commun. 10, (2019). Doi:10.1038/s41467-019-08326-7.
- X. Chen, L. Liu, and D. Shen, J. Phys. Condens. Matter 30, (2018). Doi:10.1088/1361-648X/aac523.
- M. Dendebera , T. Malyi , A. Zhyshkovych , Ya. Chornodolskyy, A. Pushak, R. Gamernyk, O. Antonyak, T. Demkiv, V. Vistovskyy, A. Voloshinovskii, "11th International Conference on Luminescent Detectors and Transformers of Ionizing Radiation" in Bydgoszcz, Poland, 2021, p. TuP1-18
- D.N. Dirin, B.M. Benin, S. Yakunin, F. Krumeich, G. Raino, R. Frison, and M. V. Kovalenko, ACS Nano 13, 11642 (2019). Doi:10.1021/acsnano.9b05481.