Journal of Physical Studies 24(1), Article 1905 [8 pages] (2020)
DOI: https://doi.org/10.30970/jps.24.1905
PHOTOIONIZATION MODELING OF PLANETARY NEBULAE WITH THE DUST GRAINS PRESENCE. II. DETERMINATION OF THE MASSES OF A NEBULAR ENVELOPE AND ITS PROGENITOR STAR USING ELECTRON TEMPERATURE IN THE CASE OF HOMOGENEOUS DISTRIBUTION OF THE NEBULAR MATTER
A. R. Kuzmak1 , B. Ya. Melekh2
1Department for Theoretical Physics, Ivan Franko National University of Lviv,
12, Drahomanov St., Lviv, UA-79005, Ukraine, e-mail: andrijkuzmak@gmail.com, 2Department for Astrophysics, Ivan Franko National University of Lviv,
8, Kyrylo and Mefodiy St., Lviv, UA-79005, Ukraine,
e-mail: bmelekh@gmail.com
Received 26 December 2019; in final form 13 February 2020; accepted 17 February 2020; published online 02 April 2020
|
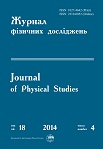 |
The study has calculated the grid of photoionization models for the homogeneous planetary nebulae in the Milky Way galaxy with the dust grain along the evolutionary tracks of their central stars. Based on these calculations, the age dependencies of the electron temperature of doubly-ionized oxygen atoms (O III) were obtained.
For nebulae with low-mass progenitor stars (up to $\approx 1.5\,M_☉$), these age dependencies contain domains with an almost constant temperature.
However, this temperature is sensitive to the masses of both the progenitor star and the nebular envelope. Thus, the behavior of the electron temperature obtained for the doubly-ionized oxygen ionization zone can be used to determine the masses mentioned above. Here we test our assumptions in the case of the Milky Way homogeneous planetary nebulae. As a result, a method for the determination of the masses of a planetary nebula envelope as well as its progenitor star using the value of the electron temperature within the doubly-ionized oxygen ionization zone is proposed. This method consists of two steps. At the first step, for a given planetary nebula we define the ratio of the emission line intensities of ionized sulfur atoms $I_{\rm [SII]λ 6716}/I_{\rm [SII]λ 6731}$. This ratio is sensitive to the age of the nebula, which allows us to obtain the limit on its age. At the second step, based on the temperature diagnostic ratio $λ 4363$\AA$/(λ 4959$\AA$ + λ 5007$\AA) between the emission line intensities of the doubly-ionized oxygen in the planetary nebula envelope, the value of the electron temperature is determined.
Taking into account the limit on the age of the planetary nebula mentioned above, the obtained result is compared with the age dependencies of the averaged electron temperature within the nebular gas for different masses of the progenitor star and the planetary nebula envelope.
Matching this value with a specific curve within the respective age range allows us to determine the masses of both the progenitor star and the planetary nebula envelope. This procedure is verified for two cases of the electron temperature determination: using the method of crossing of $n_{\rm e}-T_{\rm e}$ dependences obtained for various diagnostic ratios between emission lines as well as the popular two-zones Pagel's method (the so-called $T_{\rm e}$-method), that is based on diagnostic ratios between auroral and nebular emission lines of the doubly-ionized oxygen. As a result, using this method the mass of a progenitor star is determined with some accuracy. However, the mass of a planetary nebula envelope is determined within the accuracy of $\pm 0.1\,M_☉$.
pdf
References
- Р. РЎ. РҐСР¾Р¼Р¾РІ, РСССР¾Р½. Р¶ССР½. 39, 468 (1962).
- G. Vauclair, Ann. Astrophys. 31, 199 (1968).
- P. E. РРµССР±РµСРі, Р. РР¾СР¾РІСРєР¾РІСРєР°С, Р®. РР¾СР¾РІСРєР¾РІСРєРёР№, РР·РІ. РССР¼. Р°СССР¾СРёР·. Р¾Р±СРµСРІ. 43, 49 (1971).
- M. Perinotto, Astron. Astrophys. 39, 383 (1975).
- W. Maciel, S. R. Pottash, Astron. Astrophys. 88, 1 (1980).
- S. R. Pottash, Astron. Astrophys. 89, 336 (1980).
- J. P. Phillips, S. R. Pottash, Astron. Astrophys. 130, 91 (1984).
- S. R. Pottash, Planetary Nebulae (Reidel, Dordrecht--Boston--Lancaster, 1984).
- Р. РЎ. РҐСР¾Р¼Р¾РІ, РР»Р°Р½РµСР°СР½СРµ ССР¼Р°Р½Р½Р¾ССРё. Физика. РРІР¾Р»ССРёС. РР¾СР¼Р¾РіР¾Р½РёС (РР°СРєР°, РР¾СРєРІР°, 1985).
- C. Giovanardi, A. Natta, F. Palla, Astron. Astrophys. Suppl. Ser. 70, 269, (1987).
- B. E. J. Pagel, E. A. Simonson, R. J. Terlevich, M. G. Ed\-munds, Mon. Not. R. Astron. Soc. 255, 325 (1992);
CrossRef
- F. R. Boffi, L. Stangelini, Astron. Astrophys. 284, 248 (1994).
- D. Buckley, S. E. Schneider, Astrophys. J. 446, 279 (1995);
CrossRef
- R. A. Benjamin, E. D. Skillman, D. P. Smits, Astrophys. J. 514, 307 (1999);
CrossRef
- G. Stasińska, Yu. Izotov, Astron. Astrophys. 397, 71 (2003);
CrossRef
- V. Luridiana, A. Peimbert, M. Peimbert, M. Cervino, Astrophys. J. 592, 846 (2003);
CrossRef
- D. E. Osterbrock, G. J. Ferland, Astrophysics of Gaseous Nebulae and Active Galactic Nuclei, 2nd edition (University Science Books Sausalito, California, 2006).
- Yu. Izotov, T. Thuan, G. Stasińska, Astrophys. J. 662, 15 (2007);
CrossRef
- M. Peimbert, V. Luridiana, A. Peimbert, Astrophys. J. 666, 636 (2007);
CrossRef
- V. Luridina, Astrophys. Space. Sci. 324, 361 (2009);\linebreak
CrossRef
- V. V. Sagun, Yu. I. Izotov, Kinem. Phys. Celest. Bodies 28, 103 (2012);
CrossRef
- V. V. Holovatyy, A. V. Demchyna, J. Phys. Stud. 17, 1902 (2013).
- B. Ya. Melekh, A. V. Demchyna, V. V. Holovatyi, Kinem. Phys. Celest. Bodies 31, 73 (2015);
CrossRef
- Р. Р. РР¾Р»Р¾РІР°ССР№, Р®. Р¤. РалСРєР¾РІ, РСССР¾Р½. Р¶ССР½. 69, 1166 (1992).
- R. Szczerba, Astron. Astrophys. 181, 365 (1987).
- B. Ya. Melekh, A. R. Kuzmak, J. Phys. Stud. 16, 1902 (2012).
- Р. Р. РР¾Р»Р¾РІР°СРёР№, Р . Р. РРµССР±РµСРі, Р®. Р¤. РалСРєР¾РІ, Р. Р. РСР¾Р½РёРє, РР·РІ. РССР¼. Р°СССР¾СРёР·. Р¾Р±СРµСРІР°СР¾СРёРё 96, 72 (1999).
- Р. Р. РР¾Р»Р¾РІР°СРёР№, Р. РЇ. РелеС
, Р. Р. РавСРёР»Р¾РІР°, Р¤СР·РёРєР° СРІСССР½Р½С РіР°Р·Р¾РІРёС
ССР¼Р°Р½Р½Р¾ССей (РРР£ iР¼РµР½i IРІР°Р½Р° Р¤СР°Р½РєР°, РСРІСРІ, 2013).
- Р. Р. РЎР¾Р±Р¾Р»РµРІ, РССС СРµР¾СРµСРёСРµСРєР¾Р№ Р°СССР¾СРёР·РёРєРё (РР°СРєР°, РР¾СРєРІР°, 1967).
- Р. РЎ. РҐСР¾Р¼Р¾РІ, РР»Р°Р½РµСР°СР½СРµ ССР¼Р°Р½Р½Р¾ССРё: СРёР·РёРєР°, СРІР¾\-Р»С\-СРёС, РєР¾СР¼Р¾Р»Р¾РіРёС (РР°СРєР°, РР¾СРєРІР°, 1985).
- D. R. Garnet, Astron. J. 103, 1330 (1992).
- E. Vassiliadis, P.R. Wood, Astrophys. J. Suppl. Ser. 92, 125 (1994);
http://doi.org/10.1086/191962
- R. Tylenda, G. Stasinska, Astron. Astrophys. 228, 897 (1994).
- A. Karska, Praca magisterska na kierunku astronomia (Toruń, 2009).