Journal of Physical Studies 24(4), Article 4701 [8 pages] (2020)
DOI: https://doi.org/10.30970/jps.24.4701
MODELING OF IDEALITY FACTOR VALUE IN n+–p–p+-Si STRUCTURE
O. Ya. Olikh , O. V. Zavhorodnii
Taras Shevchenko National University of Kyiv,
64/13, Volodymyrska St., Kyiv, UA-01601, Ukraine
e-mail: olikh@univ.kiev.ua
Received 25 August 2020; in final form 19 October 2020; accepted 22 October 2020; published online 01 December 2020
|
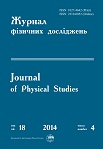 |
This paper presents the results of computer simulation of the ideality factor of silicon $n^+-p-p^+$ structure with iron contamination. The Solar Cells Capacitance Simulator (SCAPS) was the tool used for numerical simulation of these devices.
The iron concentration range of $10^{10}-10^{13}$ cm$^{-3}$, the acceptor doping level range of $10^{15}-10^{17}$ cm$^{-3}$, the temperature range of $290-340$ K, and the base thickness range of $150-240$ $μ$m were used in the investigation. The double diode model was used to extract the ideality factor.
The following cases were considered: (i) uniformly distributed lone interstitial iron atoms; (ii) coexistence of non-uniformly distributed $\mathrm{Fe}_i$ and $\mathrm{Fe}_i\mathrm{B}_s$.
It has been shown that the ideality factor value is determined by a hole occurring on the $\mathrm{Fe}_i$ level,
a trap location, and an intrinsic recombination contribution. The increase in the base thickness leads to a decrease in $n$ value.
The sign of change in the ideality factor after $\mathrm{Fe}_i\mathrm{B}_s$ dissociation depends on temperature, doping level, and iron concentration.
Key words: ideality factor, silicon, $n^+$–$p$–$p^+$ structure, SCAPS, iron concentration
Full text
References
-
K. Ishaque, Z. Salam, H. Taheri, Sol. Energy Mater. Sol. Cells 95, 586 (2011);
Crossref
-
A. J. Bühler, A. Krenzinger, Prog. Photovoltaics Res. Appl. 21, 884 (2013);
Crossref
-
O. Breitenstein, Opto-Electron. Rev. 21, 259 (2013);
Crossref
-
O. Olikh, Superlattices Microstruct. 117, 173 (2018);
Crossref
-
J. Beier, B. Voss, in Conference Record of the Twenty Third IEEE Photovoltaic Specialists Conference (1993), p. 321;
Crossref
-
K. McIntosh, P. Altermatt, G. Heiser, in 16th European Photovoltaic Solar Energy Conference: Proceedings of the International Conference and Exhibition (Glasgow, 2000), p. 250.
-
A. Kaminski et al., in Conference Record of the Twenty Fifth IEEE Photovoltaic Specialists Conference (1996), p. 573;
Crossref
-
Z. Hameiri, K. McIntosh, G. Xu, Sol. Energy Mater. Sol. Cells 117, 251 (2013);
Crossref
-
A. S. H. van der Heide, A. Schonecker, J. H. Bultman, W. C. Sinke, Prog. Photovoltaics Res. Appl. 13, 3 (2005);
Crossref
-
L. Duan et al., IEEE J. Photovoltaics 8, 1701 (2018);
Crossref
-
O. Olikh, Superlattices Microstruct. 136, 106309 (2019);
Crossref
-
A. V. Sachenko et al., Tech. Phys. Lett. 44, 873 (2018);
Crossref
-
J. Schmidt, Prog. Photovoltaics Res. Appl. 13, 325 (2005);
Crossref
-
W. Wijaranakula, J. Electrochem. Soc. 140, 275 (1993);
Crossref
-
E. Hu et al., Renew. Energy 77, 442 (2015);
Crossref
-
A. Hamache, N. Sengouga, A. Meftah, M. Henini, Radiat. Phys. Chem. 123, 103 (2016);
Crossref
-
G. Azzouzi, W. Tazibt, Energy Procedia 41, 40 (2013);
Crossref
-
R. Pässler, Phys. Rev. B 66, 085201 (2002);
Crossref
-
D. Yan, A. Cuevas, J. Appl. Phys. 116, 194505 (2014);
Crossref
-
M. A. Green, J. Appl. Phys. 67, 2944 (1990);
Crossref
-
W. O'Mara, R. Herring, L. Hant, Handbook of Semiconductor Silicon Technology
(Noyes Publications, New Jersey, 1990).
-
R. Couderc, M. Amara, M. Lemiti, J. Appl. Phys. 115, 093705 (2014);
Crossref
-
D. Klaassen, Solid-State Electron. 35, 953 (1992);
Crossref
-
R. Hull, Properties of Crystalline Silicon (Institution of Engineering and Technology, London, 1999).
-
H. T. Nguyen, S. C. Baker-Finch, D. Macdonald, Appl. Phys. Lett. 104, 112105 (2014);
Crossref
-
P. P. Altermatt, J. Schmidt, G. Heiser, A. G. Aberle, J. Appl. Phys. 82, 4938 (1997);
Crossref
-
S. Rein, S. W. Glunz, J. Appl. Phys. 98, 113711 (2005);
Crossref
-
J. D. Murphy, K. Bothe, M. Olmo, V. V. Voronkov, R. J. Falster, J. Appl. Phys. 110, 053713 (2011);
Crossref
-
H. Kohno, H. Hieslmair, A. A. Istratov, E. R. Weber, Appl. Phys. Lett. 76, 2734 (2000);
Crossref
-
F. E. Rougieux, C. Sun, D. Macdonald, Sol. Energy Mater. Sol. Cells 187, 263 (2018);
Crossref
-
B. B. Paudyal, K. R. McIntosh, D. H. Macdonald, in 34th IEEE Photovoltaic Specialists Conference (PVSC) (2009), p. 1588.
-
A. A. Istratov, H. Hieslmair, E. Weber, Appl. Phys. A Mater. Sci. Proc. 69, 13 (1999);
Crossref
-
M. Burgelman, P. Nollet, S. Degrave, Thin Solid Films 361–362, 527 (2000);
Crossref
-
K. Decock, S. Khelifi, M. Burgelman, Thin Solid Films 519, 7481 (2011);
Crossref
-
M. Cappelletti, G. Casas, A. Cédola, E. Peltzer y Blancá, B. M. Soucase, Superlattices Microstruct. 123, 338 (2018);
Crossref
-
M. Mostefaoui, H. Mazari, S. Khelifi, A. Bouraiou, R. Dabou, Energy Procedia 74, 736 (2015);
Crossref
-
C.-H. Huang, W.-J. Chuang, Vacuum 118, 32 (2015);
Crossref
-
F. Azri, A. Meftah, N. Sengouga, A. Meftah, Solar Energy 181, 372 (2019);
Crossref
-
B. Zhao, J. Zhou, Y. Chen, Physica B Cond. Mat. 405, 3834 (2010);
Crossref
-
K. Yu, J. Liang, B. Qu, X. Chen, H. Wang, Energy Convers. Manag. 150, 742 (2017);
Crossref