Journal of Physical Studies 25(4), Article 4701 [9 pages] (2021)
DOI: https://doi.org/10.30970/jps.25.4701
STRUCTURE AND ELECTRONIC PROPERTIES OF CsPbBr3 PEROVSKITE: FIRST PRINCIPLE CALCULATIONS
M. Kovalenko , O. Bovgyra , V. Kolomiets
Ivan Franko National University of Lviv, 8, Kyrylo and Mefodiy St., Lviv, UA–79005, Ukraine
Received 01 July 2021; in final form 26 October 2021; accepted 03 November 2021; published online 18 November 2021
|
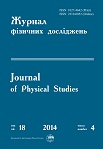 |
In recent years, inorganic cesium lead bromide (CsPbBr3) perovskite has been widely studied due to its potential spplication in light-emitting devices and solar cells. In this work, within the density functional theory framework, we performed a study of structural and electronic properties of temperature-dependent phases of the CsPbBr3 crystal, in particular, the cubic, tetragonal, and orthorhombic phases using different approximations for the exchange-correlation functional. The analysis of structural parameters shows that the lattice constants and the volume of the unit cell change when the CsPbBr3 crystal changes from the cubic phase to the tetragonal and orthorhombic structures. A good agreement between theoretical and experimental results is obtained using GGA(PBEsol) and GGA(PBEsol)+U approximations. The Mulliken population analysis indicates that between Cs and Br atoms there is a stronger ionic bonding and at the same time there is a stronger covalent bonding between Br and Pb atoms. The electronic structure of CsPbBr3 perovskite was investigated by estimating the change in the electronic properties when included in the calculations of spin-orbit coupling (SOC). The results of the calculations of the band-energy structure showed that the cubic, tetragonal, and orthorhombic crystalline phases of perovskite are semiconductors and have direct bandgaps. Also, we found that the bandgap changes with a change in the phase structure. The obtained values of the bandgap for all crystalline phases of CsPbBr3 perovskite are in good agreement with the previously obtained theoretical calculations as well as experimental data. We established that the tilting angle has a crucial effect on the bandgap width of the tetragonal and orthorhombic phases. The results of the obtained first-principle calculations show a wide temperature range of the possible use of CsPbBr3 perovskite.
\bigskip
Key words: density functional theory, bandgap, perovskite, electronic structure, density of states.
Full text
References
- G. Zhang et al., Chem. Soc. Rev. 45, 5951 (2016);
Crossref
- X. Mao et al., J. Phys. Chem. C 122, 7670 (2018);
Crossref
- G. Murtaza, I. Ahmad, Phys. B: Cond. Matt. 406, 3222 (2011);
Crossref
- M. Zhang et al., J. Cryst. Growth 484, 37 (2018);
Crossref
- H. Ito, H. Onuki, R. Onaka, J. Phys. Soc. Jpn. 45, 2043 (1978);
Crossref
- W.-Q. Liao et al., Nat. Commun. 6, 7338 (2015);
Crossref
- D.-L. Wang et al., Sci. Rep. 6, 18922 (2016);
Crossref
- K. A. Bush et al., Nat. Energy 2, 17009 (2017);
Crossref
- P. Cuiet et al., Nat. Energy 4, 150 (2019);
Crossref
- N. Yantara et al., J. Phys. Chem. Lett. 6, 4360 (2015);
Crossref
- K. Lin et al., Nature 562, 245 (2018);
Crossref
- M. Kulbak et al., J. Phys. Chem. Lett. 7, 167 (2016);
Crossref
- X. Zhang et al., ACS Appl. Mater. Interfaces 9, 4926 (2017);
Crossref
- Y. Ling et al., Adv. Mater. 28, 8983 (2016);
Crossref
- Z. Wei et al., Nanoscale 8, 18021 (2016);
Crossref
- M. Kulbak, D. Cahen, G. Hodes, J. Phys. Chem. Lett. 6, 2452 (2015);
Crossref
- V. B. Mykhaylyk et al., Sci. Rep. 10, 8601 (2020);
Crossref
- M. Rodová, J. Brožek, K. Knížek, K. Nitsch, J. Therm. Anal. Calorim. 71, 667 (2003);
Crossref
- A. S. Verma, A.Kumar, S. R. Bhardwaj, Phys. Status Solidi B 245, 1520 (2008);
Crossref
- Y. H. Chang, C. H. Park, J. Korean Phys. Soc. 44, 889 (2004).
- M. Ahmad et al., J. Alloys Compd. 705, 828 (2017);
Crossref
- R. A. Jishi, O. B. Ta, A. A. Sharif, J. Phys. Chem. C 118, 28344 (2014);
Crossref
- H. M. Ghaithan, Z. A. Alahmed, S. M. H. Qaid, M. Hezam, A. S. Aldwayyan, ACS Omega 5, 7468 (2020);
Crossref
- F. Tran, P. Blaha, Phys. Rev. Lett. 102, 226401 (2009);
Crossref
- B. Andriyevsky et al., J. Electron. Mater. 48 (9), 5586 (2019);
Crossref
- I. M. Kunyo et al., J. Phys. Stud. 22, 3301 (2018);
Crossref
- A. I. Kashuba et al., Appl. Nanosci., in press (2021);
Crossref
- M. Kovalenko et al., Phys. Chem. Solid State 22, 153 (2021);
Crossref
- I. E. Castelli, J. M. García-Lastra, K. S. Thygesen, K. W. Jacobsen, APL Mater. 28, 081514 (2014);
Crossref
- M. G. Goesten, R. Hoffmann, J. Am. Chem. Soc. 140, 12996 (2018);
Crossref
- B. Kang, K. Biswas, J. Phys. Chem. Lett. 9, 830 (2018);
Crossref
- C. C. Stoumpos et al., Cryst. Growth Des. 13, 2722 (2013);
Crossref
- Q. A. Akkerman et al., J. Am. Chem. Soc. 138, 1010 (2016);
Crossref
- R. B. Sadok, D. Hammouténe, N. Plugaru, Phys. Status Solidi B 258, 2000289 (2020);
Crossref
- J. Qian,B. Xu, W. Tian, Organ. Electron. 37, 61 (2016);
Crossref
- Ye Yuan et al., Chinese Phys. B 24, 116302 (2015);
Crossref
- Q. Chen et al., Nature 561, 88 (2018);
Crossref
- P. Giannozzi et al., J. Phys. Condens. Matter 29, 465901 (2017);
Crossref
- H. J. Monkhorst, J. D. Pack, Phys. Rev. B 13, 5188 (1976);
Crossref
- E. Welch, L. Scolfaro, A. Zakhidov, AIP Adv. 6, 125037 (2016);
Crossref
- M. S. Kirschner et al., Nat. Commun. 10, 504 (2019);
Crossref
- S. Hirotsu, J. Harada, M. Iizumi, K. Gesi, J. Phys. Soc. Jpn. 37, 1393 (1974);
Crossref
- R. Dos Reis et al., Appl. Phys. Lett. 112, 071901 (2018);
Crossref
- T. J. Whitcher et al., NPG Asia Mater. 11, 70 (2019);
Crossref
- J. Zhao et al., J. Phys. Chem. Lett. 8, 3115 (2017);
Crossref
- C. K. Moller, Nature 182, 1436 (1958);
Crossref
- K. Heidrich et al., Phys. Rev. B 24, 5642 (1981);
Crossref
- T. Paul et al., J. Mater. Chem. C 6, 3322 (2018);
Crossref
- Y. Li et al., Sol. RRL 2, 1800164 (2018);
Crossref
- O. Yaffe et al., Phys. Rev. Lett. 118, 136001 (2017);
Crossref
- G. Mannino et al., J. Phys. Chem. Lett. 11, 2490 (2020);
Crossref