Journal of Physical Studies 27(4), Article 4602 [6 pages] (2023)
DOI: https://doi.org/10.30970/jps.27.4602
QUANTUM MECHANICAL MODELING OF THE INTERACTION OF ULTRADISPERSED DIAMOND PARTICLES WITH NICKEL IONS
V. V. Tytarenko1 , V. A. Zabludovsky2
1Dnipro University of Technology, 19, Dmytra Yavornytskoho Ave. , Dnipro, UA–49005, Ukraine,
2Ukrainian State University of Science and Technologies, 2, Lazaryana St., Dnipro, UA–49010, Ukraine
Received 06 August 2023; in final form 23 October 2023; accepted 09 November 2023; published online 28 November 2023
|
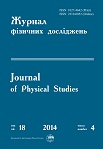 |
Composite metal coatings have been fabricated from an aqueous solution of an electrolyte that contains particles of ultradispersed diamond (UDD). Results from the cathodic polarization curve have shown an increase in charge-transfer resistance. Presumably, particles of ultradispersed diamond obtain a charge in the solution by adsorbing metal ions on its surface.
To investigate the co-deposition mechanism of metal ions and UDD particles, the authors proposed a quantum mechanical model of the interaction between nickel ions and a cell of a diamond crystal lattice corresponding to the point group of symmetry $m3m$ ($4/m$ $-3$ $2/m$). The adsorption properties of nickel atoms with the diamond cell were studied using the density functional theory method with the hybrid functional B3LYP. Models of the diamond cell with one, two, and three bound metal ions have been developed. Calculations of binding energies were performed using the GAUSSIAN 09 program package.
The obtained results for the binding energy of adsorbed nickel ions with the diamond cell confirmed the possibility of adsorption of nickel ions on the surface of UDD particles from an aqueous solution of electrolytes, leading to the formation of stable metal-carbon nanomaterial complexes. With the sequential addition of one and two metal ions to the diamond cell, the binding energies were observed to decrease from 0.200 eV to 0.126 eV. Three or more nickel ions were not retained on the surface of the diamond crystal lattice, as their binding energies were significantly lower than the thermal movement energy of 0.025 eV.
It can be assumed that the metal-carbon complexes, formed as a result of metal atom adsorption on the surface of a UDD particle, acquire a charge in the electrolyte solution and move towards the cathode under the influence of the electric field generated by the potential difference between the anode and the cathode. The results of SEM studies on the surface and metallography of end sections of electrolytic nickel coatings confirm the hypothesis regarding the co-deposition mechanism of metal ions and UDD particles on the cathode. The presence of UDD particles in the composite nickel coating is identified as dark inclusions.
Key words: metal-carbon complex, quantum mechanical model, binding energy, electrodeposition, carbon nanomaterial.
Full text
References
-
G. K. Burkat, T. Fujimura, V. Yu. Dolmatov, E. A. Orlova, M. V. Veretennikova, Diam. Relat. Mater. 14, 1761 (2005);
Crossref
-
L. Meihua et al., Materials. 12, 1105 (2019);
Crossref
-
W. Liping, G. Yan, X. Qunji, L. Huiwen, X. Tao, Mater. Sci. Eng. A 390, 313 (2005);
Crossref
-
V. P. Isakov, A. I. Lyamkin, D. N. Nikitin, A. S. Shalimova, A. V. Solntsev, Protec. Met. Phys. Chem. Surf. 46, 578 (2010);
Crossref
-
V. V. Tytarenko, V. A. Zabludovsky, E. Ph. Shtapenko, Inorg. Mater. Appl. Res. 10, 589 (2019);
Crossref
-
I. R. Robiul, Md. Hasan Ali, Md. Abu Jafor, Md. Mahmodul Alam, AIMS Mat. Sci. 6, 756 (2019);
Crossref
-
V. A. Zabludovsky, V. V. Tytarenko, E. Ph. Shtapenko, Trans. Inst. Met. Finish. 95, 337 (2017);
Crossref
-
М. Hiroshi et al., Electrochim. Acta. 52, 3047 (2007);
Crossref
-
X. He, Y. Wang, X. Sun, L. Huang, Nanosci. Nanotechnol. Lett. 4, 48 (2012);
Crossref
-
V. V. Tytarenko, V. A. Zabludovsky, E. Ph. Shtapenko, I. V. Tytarenko, Galvanotechnik 4, 648 (2019).
-
C. Gheorghies, D. E. Rusu, A. Bund, S. Condurache-Bota, L. P. Georgescu, Appl. Nanosci. 4, 1021 (2014);
Crossref
-
A. Ramanathan, P. K. Krishnan, R. Muraliraja, J. Manuf. Process. 42, 213 (2019);
Crossref
-
A. Dorri Moghadam, E. Omrani, P. L. Menezes, P. K. Rohatgi, Compos. B. Eng. 77, 402 (2015);
Crossref
-
S. R. Bakshi, D. Lahiri, A. Agarwal, Int. Mater. Rev. 55, 41 (2013);
Crossref
-
Z. Hu et al., Mater. Sci. Technol. 32, 930 (2016);
Crossref
-
S. B. Sinnott, E. C. Dickey, Mat. Sci. Eng. R. 43, 1 (2003);
Crossref
-
Q. Caihao et al., Compos. C 4, 100120 (2021);
Crossref
-
G. Cheng et al., J. Appl. Phys. 108, 123711 (2010);
Crossref
-
L. Pei, X. Jingpei, W. Aiqin, M. Dougin, M. Zhiping, Appl. Surf. Sci. 517, 146040 (2020);
Crossref
-
M. Vanin, Phys. Rev. B. 81, 081408(R) (2010);
Crossref
-
K. Pi et al., Phys. Rev. В 80, 075406 (2009);
Crossref
-
A. S. Barnard, M. Sternberg, J. Mater. Chem. 17, 4811 (2007);
Crossref
-
A. S. Barnard, J. Mater. Chem. 18, 4038 (2008);
Crossref
-
R. Skyner et al., Phys. Chem. Chem. Phys. 17, 6174 (2015);
Crossref
-
R. G. Parr, W. Yang, Density-Functional Theory of Atoms and Molecules (Oxford University Press, New York, 1989).
-
W. Koch, M. C. Holthausen, Chemists Guide to Density Functional Theory, 2nd ed. (Wiley–VCH, New York, 2001).
-
A. V. Arbuznikov, J. Struct. Chem. 48, S1 (2007);
Crossref
-
M. K. Sabbe, M. F. Reyniersa, K. Reuter, Catal. Sci. Technol. 2, 2010 (2012);
Crossref
-
N. López et al., Catal. Sci. Technol. 2, 2405 (2012);
Crossref
-
T. C. Allison, Y. Y. J Tong, Phys. Chem. Chem. Phys. 13, 12858 (2011);
Crossref
-
M. J. Frisch et al., Gaussian 09, Revision D.01 (Gaussian Inc., Wallingford CT, 2016).
-
G. Schreckenbach, P. J. Hay, R. L. Martin, Inorg. Chem. 37, 4442 (1998);
Crossref
-
G. Schreckenbach, P. J. Hay, R. L. Martin, J. Comput. Chem. 20, 70 (1999);
Crossref
-
A. D. Becke, J. Chem. Phys. 98, 5648 (1993);
Crossref
-
V. V. Tytarenko et al., J. Chem. Technol. 29, 42 (2021);
Crossref
-
V. V. Tytarenko, V. A. Zabludovsky, E. Ph. Shtapenko, I. V. Tytarenko, J. Phys. Chem. Solids State 23, 461 (2022);
Crossref