Visnyk of the Lviv University. Series Physics
56 (2019) с. 91-102
DOI: https://doi.org/10.30970/vph.56.2019.91
Simulation of the thermal stability and melting of the Ag@Pd bimetallic nanoparticle
B. Natalich, U. Shvets, V. Borysiuk
| 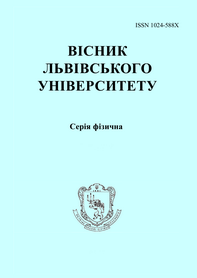 |
Within the framework of the molecular dynamics methods the simulation of the temperature stability of the metallic Ag@Pd nanoparticle with the core-shell structure was performed and the melting temperature of the sample was determined. During the simulation of the dynamic behavior of nanoparticle the calculation of forces of interatomic interactions was carried out within the embedded atom method. To simulate the melting process, the temperature of the sample was gradually increased by scaling the corresponding atomic velocities using the Berendsen thermostat in the temperature range 250--3000 К. The Lindemann index was used as a numerical parameter describing changes in the structure of the nanoparticle. According to the results of the study, the temperature dependences of the Lindeman index and the average potential energy were obtained, as well as the radial distribution functions for the Ag@Pd nanoparticle at different temperature values. The obtained dependences have a typical form: they first increase monotonically at a temperature range 250 \leq T \leq 1500 K, and when the temperature reaches about 1600 K, the Lindemann index and potential energy begin to increase rapidly, which may be considered as the beginning of the melting process. From the simulation results, atomistic configurations of the sample were built and the dynamics of changes in its structure was investigated. Spatial distribution of the atoms on Lindeman index within the volume of the sample around melting temperature was also calculated. As it is follows from the obtained data, the melting of Ag@Pd nanoparticle with spherical shape begins on the surface of the sample, as well as in a core which consists of Ag atoms. Calculated data allowed us to determine the temperature where destruction of the crystalline structure of the sample occurs. Melting behavior of nanoparticles with different ratios of Ag and Pd concentrations and diameters are also studied.
Full text (pdf)
References
- S. J. Mejia-Rosales C. Fernandez-Navarro and E. Perez-Tijerina, Phys. Chem. B. 110(26), 12884--12889(2006), https://doi.org/10.1021/jp0614704.
- M. Tsuji N. Miyamae and S. Lim, Cryst. Growth Des. 6(8), 1801--1807(2006), https://doi.org/10.1021/cg060103e.
- S.--W. Baek G. Park and J. Noh, ACS Nano. 8(4), 3302--3312(2014), https://doi.org/10.1021/nn500222q.
- R. Gh. Chaudhuri and S. Paria, Chem. Rev. 112(4), 2373--2433(2012), https://doi.org/10.1021/cr100449n.
- І. М. Мудрак Л. П. Сторожук and С. М. Махно, Наносистеми, наноматеріали, нанотехнології. 10(4), 819--827(2012).
- W. I. Abdel-Fattah M. M. Eid and Sh. I. Abd El-Moez, Life Sci. 183, 28--36(2017), https://doi.org/10.1016/j.lfs.2017.06.017.
- S. Alayoglu F. Tao and V. Altoe, Catal. Lett. 141(5), 633--640(2011), https://doi.org/10.1007/s10562-011-0565-7.
- W.-Y. Yu G. M. Mullen and D. W. Flaherty, J. Am. Chem. Soc. 136(31), 11070--11078(2014), https://doi.org/10.1021/ja505192v.
- O. Metin H. Can and K. Sendil, J. Colloid Interface Sci. 498, 378--386(2017), https://doi.org/10.1016/j.jcis.2017.03.066.
- K. Tedsree T. Li and S. Jones, J. Nat. Nanotech. 6, 302--307(2011), https://doi.org/10.1038/nnano.2011.42.
- С. П. Туранская А. Д. Четыркин and И. В. Дубровин, Поверхность. 3, 343--366(2011).
- W. I Abdel-Fattah A. S. M. Sallam and N. A. Attawa, Materials Research Express. 1(3), 35024(2014), https://doi.org/10.1088/2053-1591/1/3/035024.
- D. Ch. Ch. Li H. Liu and F. Ye, Sci Rep-UK. 5, 11949(2015), https://doi.org/10.1038/srep11949.
- T. Zhanga L. Li and Zh. Yea, RSC Adv. 8, 18252--18259(2018), https://doi.org/10.1039/C8RA02563K.
- B. Zhang Y. Yuan and K. Ph. N. Yan, Catal. Sci. Technol. 5, 1683--1692(2015), https://doi.org/10.1039/C4CY01382D.
- H. A. Alarifi M. Atis and C. Ozdogan, J. Phys. Chem. C. 117(23), 12289--12298(2013), https://doi.org/10.1021/jp311541c.
- Zh. Yang X. Yang and Zh. Xu, J. Phys. Chem. C. 112(13), 4937--4947(2008), https://doi.org/10.1021/jp711702y.
- H. H. Kart H. Yildirim and S. O. Kart, Mat. Chem. Phys. 147(1--2), 204--212(2014), https://doi.org/10.1016/j.matchemphys.2014.04.030.
- Sh. Lu J. Zhang and H. Duan, Chem. Phys. 363(1--3), 7--12(2009), https://doi.org/10.1016/j.chemphys.2009.06.010.
- R. Essajai and N. Hassanain, J. Mol. Liq. 261, 402--410(2018), https://doi.org/10.1016/j.molliq.2018.04.051.
- A. Stukowski, Modelling Simul. Mater. Sci. Eng. 18(1), 15012(2010), https://doi.org/10.1088/0965-0393/18/1/015012.
- H. J. C. Berendsen J. P. M. Postma and W. F. van Gunsteren, J.Chem. Phys. 81 (8), 3684 (1984), https://doi.org/10.1063/1.448118.
- M. I. Baskes, Phys. Rev. B. 46(5), 2727(1992), https://doi.org/10.1103/PhysRevB.46.2727.
- S. Plimpton, J. Comput. Phys. 117(1), 1--19(1995), https://doi.org/10.1006/jcph.1995.1039.
- K. Zhang G. M. Stocks and J. Zhong, Nanotechnology. 18(28), 285703(2007), https://doi.org/10.1088/0957-4484/18/28/285703.
- D. C. Rapaport, The art of molecular dynamics simulation, 1st ed. (NY: Cambridge University Press, 2004).
- C. R. Hammond, The Elements, in Handbook of Chemistry and Physics, 81st edition. (CRC press, 2000).