Journal of Physical Studies 25(3), Article 3901 [11 pages] (2021)
DOI: https://doi.org/10.30970/jps.25.3901
CONTINUOUS APPROXIMATIONS FOR LONG-TERM NUMERICAL SIMULATIONS OF THE SOLAR SYSTEM
S. Rehman
Department of Mathematics, University of Engineering and Technology, Lahore, Pakistan,
srehman@uet.edu.pk
Received 16 January 2021; in final form 28 July 2021; accepted 26 August 2021; published online 30 September 2021
|
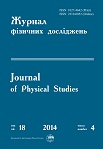 |
We present and analyse the performance of different combinations of four higher-order numerical integrators and up to nine interpolation schemes applied to the problem involving the Sun and four Gas-giants (outer planets), namely, Jupiter, Saturn, Uranus, and Neptune. The Hermite interpolation schemes obtained by one, two, and three time-step and interpolants for ODEX2 and ERKN integrators are considered in this paper. The interpolants are a special example of an interpolation scheme, which produce an approximation that is continuous across one step and across the complete interval of integration. The interpolants are quite expensive in comparison with the other interpolation schemes. Therefore, one of the objectives of this paper is to investigate the possibilities of replacing the interpolants of certain integrators by other interpolation schemes, perhaps at a cost of a little bit of accuracy. The experiments are performed to examine the error growth in the positions, velocities, and relative error in energy and angular momentum using different combinations of integrators and interpolation schemes over a long interval of integration, as long as 100 million years for the Jovian problem with local error tolerances ranging from $10^{-16}$ to $10^{-08}$.
Key words: Jovian problem, interpolation schemes, long-term simulations
Full text
References
- T. S. Baker, J. R. Dormand, P. J. Prince, App. Num. Math. 29, 171 (1999);
Crossref
- J. Dormand, M. E. A. El-Mikkawy, P. Prince, J. Numer. Anal. 7, 423 (1987);
Crossref
- J. R. Dormand, P. J. Prince, Celest. Mech. 18, 223 (1978);
Crossref
- J. R. Dormand, P. J. Prince, Comp. Math. Appl. 12A, 1007 (1986);
Crossref
- J. R. Dormand, P. J. Prince, Comp. Math. Appl. 13, 937 (1987);
Crossref
- W. H. Enright, K. R. Jackson, S. P. Norsett, P. G. Thomsen, ACM Trans. Math. Softw. 12, 193 (1986);
Crossref
- K. R. Grazier, W. I. Newman, P. W. Sharp, Astron. J. 145, 112 (2013):
Crossref
- K. R. Grazier, W. I. Newman, J. M. Hyman, P. W. Sharp, ANZIAM J. 46, C1086 (2005);
Crossref
- K. R. Grazier, W. I. Newman, W. M. Kaula, J. M. Hyman, Icarus 140, 341 (1999);
Crossref
- E. Hairer, S. P. N{\o}rsett, G. Wanner, Solving Ordinary Differential Equations I: Nonstiff Problems (Springer-Verlag, 1987).
- M. K. Horn, SIAM J. Num. Anal. 20, 558 (1983);
Crossref
- E. J. Nyström, Acta Soc. Sci. Fennicae 50, 1 (1925);
https://lib.ugent.be/catalog/rug01:001785116
- L. F. Shampine, SIAM J. Num. Anal. 22,1014 (1985);
Crossref
- C. Störmer, Radium (Paris) 9, 395 (1912);
Crossref
- Ch. Tsitouras, G. Papageorgiou, Computing 43, 255 (1990);
Crossref
- J. H. Verner, SIAM J. Numer. Anal. 30, 1446 (1990);
Crossref
- S. Rehman, J. Comput. Appl. Math. 4, 446 (2014);
Crossref
- K. R. Grazier, W. I. Newman, F. Varadi, W. M. Kaula, J. M. Hyman, Icarus 140, 353 (1999);
Crossref
- D. R. Kirsh, M. Duncan, R. Brasser, H. F. Levison, Icarus 199, 197 (2009);
Crossref
- M. S. Tiscareno, R. Malhotra, Astron. J. 138, 827 (2009);
Crossref
- P. S. Lykawka, J. Horner, B. W. Jones, T. Mukai, Month. Not. R. Ast. Soc. 4, 1715 (2009);
Crossref
- D. A. Minton, R. Malhotra, Icarus 207, 744 (2010);
Crossref
- P. W. Sharp, ACM Trans. Math. Softw. 32, 375 (2006);
Crossref